Translate this page into:
Impact of the gut–brain axis on neurodegenerative diseases and potential benefits of probiotics
Corresponding author: Dr. Saptadip Samanta, Department of Physiology, Midnapore College, Raja Bazar, Midnapore, India. saptadip174@gmail.com
-
Received: ,
Accepted: ,
How to cite this article: Samanta S, Parua Mondal S. Impact of the gut–brain axis on neurodegenerative diseases and potential benefits of probiotics. South Asian J Health Sci. 2024;1:56–61. doi: 10.25259/SAJHS_17_2023
Abstract
Gut microbiota are an essential community for human health. They produce different bioactive components and short-chain fatty acids for physiological benefits. Intestinal flora elicits an immune response and maintains a balance between inflammatory and anti-inflammatory cytokines during the inflammatory response. The intricate relationship between the activities of gut microbes and brain functions creates the gut–brain axis. Microbial metabolites of the gut reach the brain through blood and lymphatic circulation. The bioactive components from gut microorganisms regulate oxidative stress, cytokine profiles, neuroinflammation, apoptotic reactions and neurodegeneration. Dysbiosis alters the activity of gut microbiota, which causes gastrointestinal disorders, neurodegenerative diseases, metabolic syndrome and cancers. Probiotic bacteria (Lactobacillus spp., Bifidobacterium spp.) have different health promotional effects and exhibit a significant role in immunomodulation. These bacteria display some protective role against various diseases, including neurodegenerative disorders.
Keywords
Gut microbiota
Gut–brain axis
Neurodegenerative diseases
Probiotics
Short chain fatty acids
INTRODUCTION
The human gastrointestinal (GI) tract, particularly the lower intestine, is the common habitat of microorganisms. This area forms an interface between microbes and intestinal epithelial cells. This colonised form of microbial population in the GI tract is called the gut microbiota, which comprise bacteria, archaea, viruses, fungi, protozoa and helminths.[1,2] Among the microorganisms, bacteria are predominant and approximately 3 × 1013 bacteria are present in the gut.[3] The common phyla of bacterial populations are Firmicutes, Bacteroidetes, Actinobacteria, Proteobacteria and Fusobacteria. Gut microbiota have several physiological benefits. They ferment indigestible sugar to produce B vitamins (B1, B2, B6, biotin, folic acid and B12), vitamin K, short-chain fatty acids (SCFAs), indole derivatives, phenolic compounds and polyamines. Gut microorganisms inhibit the colonisation and proliferation of pathogenic bacteria. SCFAs show immunomodulatory and anti-inflammatory effects.
Alteration in the gut microbial population (abundance of pathogenic organisms) causes dysbiosis. Several factors, such as chronic GI tract infection, antibiotic treatment, dietary impairment and ageing, increase the risk of dysbiosis. Dysbiosis promotes the occurrence of different diseases, including gastritis, diarrhoea, ulceration, inflammatory bowel disease (IBD), obesity, metabolic disorders, diabetes, cardiovascular diseases, GI and extra-GI cancers, allergic reactions, depression and neurodegenerative diseases.[4]
The connection between the brain and GI functions was first established in the classical experiment of Pavlov. Later, several articles exhibit the bidirectional routes of gut–brain association.[5-8] The useful relationship between gut microbial activities and brain functions forms the gut–brain axis (GBA). The effective role of intestinal microflora on brain functions starts during intrauterine life. Gut microbiota help in the maturation and development of the brain. GBA regulates neuroendocrine functions, metabolic activities, brain functions and cognitive behaviour. Germ-free animals or potent antibiotic treatment show neurochemical changes and impaired brain functions.[9] GBA potentially regulates neuroimmune functions. Dysbiosis influences oxidative stress (OS), inflammatory response and neurodegeneration.
Probiotic organisms (Lactobacillus spp., Bifidobacterium spp) prevent dysbiosis and protect the GI tract from pathogenesis. Several prebiotic substances like inulin, fructooligosaccharides, galactooligosaccharides, resistant starch and pectin influence the growth of probiotic bacteria and increase the beneficial effects. Fermented food and drinks, and beverages are rich sources of probiotic bacteria. They produce SCFAs and other beneficial metabolites to prevent inflammatory reactions and neurodegenerative diseases. The present review focuses on the role of GBA in the development of neurodegenerative diseases, such as multiple sclerosis (MS), Parkinson’s disease (PD) and Alzheimer’s disease (AD), and the impacts of probiotic bacteria as the protective factor.
THE GBA
The bidirectional relationship between gut microbiota and the central nervous system (CNS) is recognised as the GBA [Figure 1]. Gut microbiota has a role in the maturation and development of the human CNS.[10] The functions of the autonomic nervous system (ANS), and the hypothalamic-pituitary-adrenal (HPA) axis as well as the enteric nervous system (ENS), are associated with intestinal flora.[11] The metabolic products of gut microbiota have important roles in neurogenesis, immunomodulatory effects and neural activities. Improper lifestyle, stress and faulty food habits alter GI functions, metabolic activities and neuroendocrine functions. Collectively, these effects can modify the distribution pattern and functional aspects of the gut microbiota.[12,13] On the other hand, Smith[9] reported that any impairment in gut microbiota altered the activity of CNS. Gut microbiota has a role in neurogenesis. They regulate the hippocampal neurogenesis for the progression of the learning and memory process.[14]
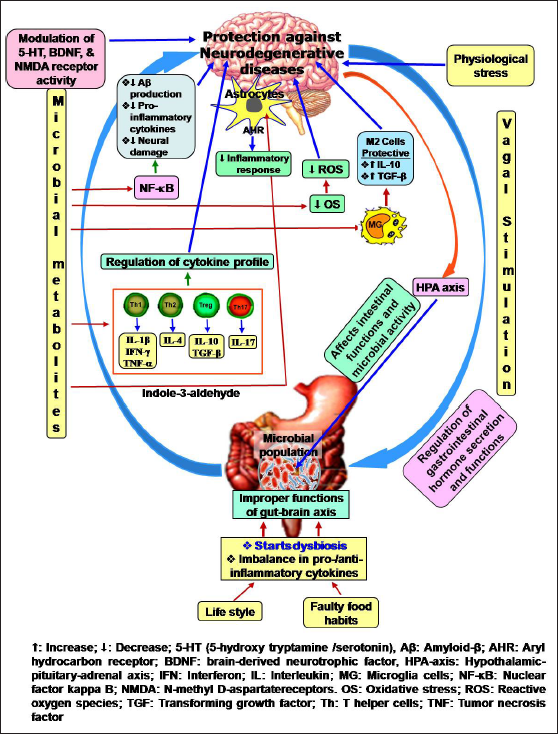
- Functions of gut–brain axis and impacts of dysbiosis.
Gut microbiota have different effects on the host’s metabolism and synthesis of neuroactive compounds that modulate neural functions, plasticity and behaviour. Gut bacteria also help in the maintenance of the integrity of the blood–brain barrier. Any impairment in the blood–brain barrier promotes gliomagenesis. Disruption of the blood–brain barrier increases the infiltration of immune cells in the brain tissue. On the other hand, the improper activity of gut microbiota changes the functions of the host immune system and cytokine release.
A higher degree of inflammation occurs during dysbiosis. Inflammatory response increases OS, leukocyte infiltration, cytokine release and activation of the signalling pathways. Proinflammatory cytokines, such as interleukin-1β (IL-1β), IL-6, IL-8, IL-12, and tumour necrosis factor-α (TNF-α) promote chronic inflammation and induce the production of reactive oxygen species (ROS). Chronic inflammation and ROS are crucial factors for neurodegeneration. The experimental study of Smith revealed that germ-free animals or any impairment in gut microbiota in animals altered the functions of CNS.[9] The germ-free animals exhibit problems in higher brain functions like poor learning and memory formation, improper emotion and behaviour.[15] These types of impairments are associated with microbiome-induced alteration in neuro-chemistry. The gut microbiomes change the activity of 5-HT (5-hydroxytryptamine/serotonin), BDNF (brain-derived neurotrophic factor) and NMDA (N-methyl D-aspartate) receptors. Thus, dysbiosis not only starts GI diseases but also intensifies CNS disorders.
The vagus is the most important nerve in the GI system. It regulates motility and secretory functions of the GI tract. This nerve maintains the gut–brain functions bi-directionally. Any dysfunction in the vagal system promotes mood disorder, neurodegenerative diseases, GI pathogenesis and IBD.[16] Microbial metabolites increase the secretion of 5-HT, cholecystokinin (CCK) and peptide YY is the name of a protein from hormone-secreting GI cells through TLR (toll-like receptor) response. These factors activate the vagal afferent and complete the gut–brain circuit.[17,18]
Macrophages, CD8+, Treg and CD4+ (CD: cluster of differentiation) T-helper cells are involved in immunity. Lymphocytes cross the blood–brain barrier and are distributed in the CNS. Gut microbiota activate the immune-competent cells. Bacteroides fragilis activates Th1 through polysaccharide A. Segmented filamentous bacteria Acinetobacter baumannii and Porphyromonas uenonis can activate Th17 cells.[8] The IFN-γ-producing Th1 cells induce MS while IL-4-producing Th2 cells exert a protective role.[19] Kleinewietfeld and Hafler reported that Foxp3-expressing Treg cells produced anti-inflammatory cytokines IL-10 and TGF-β. Clostridium stimulates the development of Treg cells.[20] Therefore, gut microbiota always maintain a balance among the immune-competent cells.
The microglia cells are present throughout the CNS. They are involved in synaptic transmission and neural circuit formation.[21] These cells also protect the brain from pathogenesis and regulate phagocytosis, inflammation and cytokine production.[22] Microglia cells are in two states, pro-destructive (M1 microglia) and tissue-regenerating (M2 microglia). This process is interconvertible. In response to the pathogenic challenges, microglia stimulate T cells for the secretion of TNF-α, IL-6 and IL-1β as the proinflammatory cytokines. On the other hand, T cells also secrete IL-4 and IL-13 that help to transform the M1 microglia to M2 microglia, resulting in a diminution of inflammatory response. Impaired stimulation and activity of M1 and M2 microglia cells can promote tumour formation in the brain.[23] Gut microbiota regulate the maturation and activity of microglia cells. The germ-free animals or antibiotic-treated animals showed a large number of naïve microglia cells and fewer mature microglia cells, resulting in functional impairment [Figure 1].[8]
Astrocytes are abundantly present in the CNS. They maintain the integrity of the blood–brain barrier, ionic balance, perfusion ratio and nutrient supply.[24] Gut microbial metabolite indole-3-aldehyde [aryl hydrocarbon receptors (AHR) agonist] is synthesised from tryptophan and can bind with the AHR of astrocytes. This binding prevents inflammatory response through IFN-I signalling [Figure 1].[25] The metabolites of gut microbiota elevate the expression of occludin and claudin-5 to increase the strength of tight junction and the integrity of the blood–brain barrier.[26] Boillot et al. reported that oral microbes Porphyromonas gingivalis activated the astrocytes by TLR4 for the induction of chronic inflammation.[27]
EFFECTS OF THE GBA ON NEUROLOGICAL DISORDERS
Role in Multiple sclerosis (MS)
MS is a neuro-inflammatory disease. Demyelination occurs due to an inflammatory response that leads to motor impairment, sensory loss, visual defects, autonomic dysfunction and cognitive failure.[28] Overactivation of Th1 and Th17 cells occurs in MS. The presence of fewer number of Treg cells is a common phenomenon in MS patients.[29] Gut microbiota crucially protect MS patients. The intestinal microorganisms Akkermansia muciniphila and Acinetobacter calcoaceticus are positively correlated with MS patients. They synthesise SCFAs as fermentative by-products. SCFAs activate GPR-inflammasome-dependent activity, which enhances the differentiation of Treg cells by modulating the activity of histone acetylation and deacetylation in Foxp3+ cells.[8] This inductive activity augments the differentiation of Treg cells.[30] SCFAs also inhibit the formation of inflammation-induced Th17 and IL-17 secretion. Thus, SCFAs-mediated anti-inflammatory activity potentially prevents MS. Dietary long-chain fatty acids activate Th1 and Th17 cells, leading to enhanced production of IL-17, TNF-α and IFN-γ. All these factors have direct effects on the brain and contribute to MS.[31]
Parkinson’s disease (PD)
PD is a common neurodegenerative disorder in which dopaminergic neurons are declining in the substantia nigra followed by the accumulation of α-synuclein and deposition of Lewy bodies in the remaining neurons.[32] Stokholm et al. revealed that α-synucleinopathy was started in the ENS at the early stage of the disease before the onset of complications in the CNS.[33] Alpha-synuclein overexpressing microbiota in mice significantly advances PD.[34] The abundant presence of certain species of Enterobacteriaceae and the lack of Lachnospiraceae in the gut profoundly aggravate PD.[8] At the substantia nigra of basal ganglia, TLRs (TLR4 and TLR2) bind with misfolded α-synuclein and trigger the production of proinflammatory cytokines in the neural tissue. The TLR2 specifically activates MyD88 and nuclear factor kappa B (NF-κB) signalling pathways and increases the levels of TNF and IL-1β for the progression of parkinsonism.[35,36]
Alzheimer’s disease (AD)
AD is a chronic neurodegenerative disease with subsequent loss of learning and memory. AD is characterised by the deposition of amyloid beta (Aβ) peptide outside neurons while phosphorylated tau protein accumulates inside the neuron. Aβ and phosphorylated tau dampen synaptic activity and destabilise microtubule and Ca2+ homeostasis. Aβ also triggers neuronal apoptosis.[8,37] Significant reduction of gut bacteria Ruminococcus and Butyricicoccus diminishes the production of SCFAs and advances AD.[38] Minter et al. observed that antibiotic treatment in the murine system caused dysbiosis that amplified beta amyloidosis and neuroinflammation in AD.[39] Aβ induces neuroinflammation, ROS production, inducible nitric oxide synthase (iNOS) and cyclooxygenase-2 (COX-2) activation and NF-κB signalling, resulting in the progression of neuro-degeneration in AD [Figure 1].[40] SCFAs have a protective role against inflammatory response. Insufficient SCFAs synthesis during dysbiosis or the presence of long-chain fatty acids in diet increases the risk of inflammation and development of AD.
Neuroinflammation is one of the crucial reasons for neurodegenerative diseases. Several researches on PD and AD models are trying to explore the effect of GBA and the significant role of probiotics for the treatment of AD, PD, and other diseases. GBA plays a crucial role for brain development in early stage life and prevention of neurodegeneration in aged people.
IMPACTS OF PROBIOTIC ORGANISMS ON NEURODEGENERATION
Beneficial effects of probiotic organisms and their metabolites
Probiotic is a live microbial feed supplement, which has several health benefits in the host.[41–46] Most of the probiotic organisms belong to the genera of Lactobacilli and Bifidobacteria. Despite these, other organisms such as Lactococci and Streptococci, a few species of Bacillus, Propionibacterium and Saccharomyces are also considered probiotic organisms. Supplementation of prebiotics stimulates the growth of the beneficial bacteria that start the fermentation of prebiotic components for the synthesis of beneficial agents. Probiotic bacteria increase the synthesis of bioactive compounds and SCFAs. These components reach the CNS through the circulatory system and prevent neurodegeneration. Probiotic organisms produce indole derivatives like indole-3-propionic acid (IPA) that have antioxidant properties, resulting in a reduction of OS and ROS production. Reduction of ROS diminishes the risk of neurodegeneration. IPA also reduces Aβ synthesis and prevents AD. Moreover, IPA decreases TNF-α release and inflammatory response by activating pregnane X receptors. Lactobacilli can produce indole-3-aldehyde from tryptophan that binds with AHR, leading to suppression of inflammatory response.[47] Phenolic compounds [4-OH phenylacetic acid, equol, urolithins, 8-prenylnaringenin, 2-(3,4-dihydroxy phenyl) acetic acid, 3-(4-hydroxyphenyl) propionic acid and 5-(3,4-dihydroxyphenyl) valeric acid] from gut bacteria decrease OS and platelet aggregation. These effects resist circulatory defects and reduce the chance of stroke. Probiotic bacteria maintain the synthesis of polyamines (putrescine, spermidine and spermine). Polyamines induce the synthesis of junctional proteins. Spermine reduces proinflammatory cytokine release by inhibiting the activity of M1 macrophages.
Effects on inflammatory response
Probiotic bacteria control the release of inflammatory cytokines. These bacteria protect the inhibitor of kappa B (IκB) from degradation. They induce the activity of peroxisome proliferator activator receptor γ (PPARγ) and block the action of RelA to inhibit the translocation of NF-κB to the nucleus. Inhibition of NF-κB activity decreases the expression of proinflammatory cytokines as well as inflammatory response. Probiotic organisms induce IL10 production from Th2 and macrophages. They also decrease IFN-γ and TNF-α secretion. Acetate (C2), propionate (C3) and butyrate (C4) are the major SCFAs. They promote the maturation of Treg cells to balance immune activity. Acetate promotes the development of Treg cells from naïve CD4+ T cells. Propionate acts through G-protein coupled receptor 43 (GPR-43) of FoxP3+ cells for the development of Treg cells. They suppress inflammatory response by inducing the secretion of IL-10. Butyrate acts as histone deacetylase (HDAC) and restricts IL-12 and IL-6 expression. Butyrate activates PPAR γ, which inhibits STAT, AP-1 and NF-κB-mediated activity. NF-κB is a potent transcription factor that induces the expression of inflammatory cytokines. Inhibition of NF-κB activity significantly decreases inflammatory response. It has already been discussed that inflammatory response is the prime factor for neurodegenerative diseases. Although OS, neuroinflammation and metabolic impairment have a potential role in neurodegenerative diseases, prevention of inflammatory response protects the brain from OS, synaptic disintegration and apoptotic reaction. Thus, probiotic bacteria play a positive role in preventing neurodegeneration. Moreover, probiotic bacteria have several other benefits. They show anti-cancer effects and prevent gastric, hepatic, colorectal, breast and other cancers.[4] Consumption of probiotics restricts urinary tract infection.[48] Probiotic organisms resist metabolic diseases like obesity and diabetes.[49] Moreover, maintenance of proper lifestyle, food habits and consumption of probiotic-rich foods seem to be the most beneficial for preventing neurodegenerative diseases.
CONCLUSION
Recently, neurodegenerative diseases have been a serious problem globally, and there are several causes for these diseases, including sleep disorders, OS, neuroinflammation, circadian rhythm dysfunction and others. The GBA is an essential connection for the regulation of brain functions and also protects the brain from neurodegeneration. Neuroinflammation is one of the critical factors in neurodegenerative diseases. Microbial metabolites maintain brain health and regulate the inflammatory response that protects the neurons from apoptosis and degeneration. Physiological stress and dysbiosis disturb the functions of GBA and increase the risk of neurodegenerative diseases. Circadian rhythm dysfunction is another factor that influences neurodegenerative diseases in different ways. It disrupts the activity of gut microbiota and exerts negative impacts on GBA. Therefore, multidimensional research is essential to explore the importance of probiotics in the protection of neurodegenerative diseases. Moreover, sufficient clinical trials are required to establish the benefits of probiotic bacteria against neurological disorders. Thus, integrative research can open a new arena to fight against neurodegenerative diseases.
Acknowledgment
The authors are grateful to Midnapore College, Midnapore, West Bengal, India, for providing all kinds of facilities to prepare this manuscript.
Ethical approval
Institutional Review Board approval is not required.
Declaration of patient consent
Patient’s consent is not required as there are no patients in this study.
Financial support and sponsorship
Nil.
Conflicts of interest
There are no conflicts of interest.
Use of artificial intelligence (AI)-assisted technology for manuscript preparation
The authors confirm that there was no use of artificial intelligence (AI)-assisted technology for assisting in the writing or editing of the manuscript and no images were manipulated using AI.
References
- Study insights into gastrointestinal cancer through the gut microbiota. BioMed Res Int. 2019;2019:8721503.
- [CrossRef] [PubMed] [PubMed Central] [Google Scholar]
- Gut microbiota and cancer: From pathogenesis to therapy. Cancers. 2019;11:38.
- [CrossRef] [PubMed] [PubMed Central] [Google Scholar]
- Clinical applications of gut microbiota in cancer biology. Semin Cancer Biol. 2019;55:28-36.
- [CrossRef] [PubMed] [Google Scholar]
- Potential impacts of prebiotics and probiotics on cancer prevention. Anticancer Agents Med Chem. 2022a;22:605-28.
- [CrossRef] [PubMed] [Google Scholar]
- The gut-brain axis: Interactions between enteric microbiota, central and enteric nervous systems. Ann Gastroenterol. 2015;28:203-09.
- [PubMed] [PubMed Central] [Google Scholar]
- Integrated neural and endocrine control of gastrointestinal function. Adv Exp Med Biol. 2016;891:159-73.
- [CrossRef] [PubMed] [Google Scholar]
- From the bottom-up: Chemotherapy and gut-brain axis dysregulation. Front Behav Neurosci. 2018;12:104.
- [CrossRef] [PubMed] [PubMed Central] [Google Scholar]
- Impact of microbiota on central nervous system and neurological diseases: The gutbrain axis. J Neuroinflammation. 2019;16:53.
- [CrossRef] [PubMed] [PubMed Central] [Google Scholar]
- The tantalizing links between gut microbes and the brain. Nature. 2015;526:312-14.
- [CrossRef] [PubMed] [Google Scholar]
- The central nervous system and the gut microbiome. Cell. 2016;167:915-32.
- [CrossRef] [PubMed] [PubMed Central] [Google Scholar]
- The neuroendocrinology of the microbiota-gut-brain axis: A behavioural perspective. Front Neuroendocrinol. 2018;51:80-101.
- [CrossRef] [PubMed] [Google Scholar]
- Microbial endocrinology: Host-bacteria communication within the gut microbiome. J Endocrinol. 2015;225:R21-34.
- [CrossRef] [PubMed] [Google Scholar]
- Stress & the gut-brain axis: Regulation by the microbiome. Neurobiol Stress. 2017;7:124-36.
- [CrossRef] [PubMed] [PubMed Central] [Google Scholar]
- Neurogenesis-mediated forgetting minimizes proactive interference. Nat Commun. 2016;7:10838.
- [CrossRef] [PubMed] [PubMed Central] [Google Scholar]
- Microbial memories: Sex-dependent impact of the gut microbiome on hippocampal plasticity. Eur J Neurosci. 2021;54:5235-44.
- [CrossRef] [PubMed] [PubMed Central] [Google Scholar]
- The vagus nerve in the neuro-immune axis: Implications in the pathology of the gastrointestinal tract. Front Immunol. 2017;8:1452.
- [CrossRef] [PubMed] [PubMed Central] [Google Scholar]
- Gut chemosensing mechanisms. J Clin Invest. 2015;125:908-17.
- [CrossRef] [PubMed] [PubMed Central] [Google Scholar]
- Enteroendocrine cells: Metabolic relays between microbes and their host. Endocr Dev. 2017;32:139-64.
- [CrossRef] [PubMed] [Google Scholar]
- Impacts of microbiome metabolites on immune regulation and autoimmunity. Immunology. 2018;154:230-8.
- [CrossRef] [PubMed] [PubMed Central] [Google Scholar]
- Regulatory T cells in autoimmune neuroinflammation. Immunol Rev. 2014;259:231-44.
- [CrossRef] [PubMed] [PubMed Central] [Google Scholar]
- Microglia function in central nervous system development and plasticity. Cold Spring Harb Perspect Biol. 2015;7:a020545.
- [CrossRef] [PubMed] [PubMed Central] [Google Scholar]
- Microglia development and function. Annu Rev Immunol. 2014;32:367-402.
- [CrossRef] [PubMed] [PubMed Central] [Google Scholar]
- The gut–brain aaxis, paving the way to brain cancer. Trends Cancer. 2019;5:200-7.
- [CrossRef] [PubMed] [PubMed Central] [Google Scholar]
- Diversity of astrocyte functions and phenotypes in neural circuits. Nat Neurosci. 2015;18:942-52.
- [CrossRef] [PubMed] [PubMed Central] [Google Scholar]
- Type I interferons and microbial metabolites of tryptophan modulate astrocyte activity and central nervous system inflammation via the aryl hydrocarbon receptor. Nat Med. 2016;22:586-97.
- [CrossRef] [PubMed] [PubMed Central] [Google Scholar]
- The gut microbiota influences blood-brain barrier permeability in mice. Sci Transl Med. 2014;6:263ra158.
- [CrossRef] [PubMed] [PubMed Central] [Google Scholar]
- Periodontal microbiota and phospholipases: the oral infections and vascular disease epidemiology study (INVEST) Atherosclerosis. 2015;242:418-23.
- [CrossRef] [PubMed] [PubMed Central] [Google Scholar]
- Multiple sclerosis: pathology, diagnosis and treatments. Exp Ther Med. 2017;13:3163-6.
- [CrossRef] [PubMed] [PubMed Central] [Google Scholar]
- Multiple sclerosis patients have reduced resting and increased activated CD4+CD25+FOXP3+T regulatory cells. Sci Rep. 2021;11:10476.
- [CrossRef] [PubMed] [PubMed Central] [Google Scholar]
- Role of short chain fatty acids in controlling Tregs and immunopathology during mucosal infection. Front Microbiol. 2018;9:1995.
- [CrossRef] [PubMed] [PubMed Central] [Google Scholar]
- Dietary fatty acids directly impact central nervous system autoimmunity via the small intestine. Immunity. 2016;44:951-53.
- [CrossRef] [PubMed] [Google Scholar]
- Neuropathology of genetic synucleinopathies with parkinsonism: Review of the literature. Mov Disord. 2017;32:1504-23.
- [CrossRef] [PubMed] [PubMed Central] [Google Scholar]
- Pathological alpha-synuclein in gastrointestinal tissues from prodromal Parkinson disease patients. Ann Neurol. 2016;79:940-9.
- [CrossRef] [PubMed] [Google Scholar]
- Gut microbiota regulate motor deficits and neuroinflammation in a model of Parkinson’s disease. Cell. 2016;167:1469-1480.e12 e1412.
- [CrossRef] [PubMed] [PubMed Central] [Google Scholar]
- Selective targeting of the TLR2/MyD88/NF-κB pathway reduces α-synuclein spreading in vitro and in vivo. Nat Commun. 2021;12:5382.
- [CrossRef] [PubMed] [PubMed Central] [Google Scholar]
- Activation of MyD88-dependent TLR1/2 signaling by misfolded alpha-synuclein, a protein linked to neurodegenerative disorders. Sci Signal. 2015;8:ra45.
- [CrossRef] [PubMed] [PubMed Central] [Google Scholar]
- Molecular and cellular mechanisms underlying the pathogenesis of Alzheimer’s disease. Mol Neurodegener. 2020;15:40.
- [CrossRef] [PubMed] [PubMed Central] [Google Scholar]
- Altered gut microbiota in a mouse model of Alzheimer’s disease. J Alzheimers Dis. 2017;60:1241-57.
- [CrossRef] [PubMed] [Google Scholar]
- Antibiotic-induced perturbations in gut microbial diversity influences neuro-inflammation and amyloidosis in a murine model of Alzheimer’s disease. Sci Rep. 2016;6:30028.
- [CrossRef] [PubMed] [PubMed Central] [Google Scholar]
- Microglia, neuroinflammation, and beta-amyloid protein in Alzheimer’s disease. Int J Neurosci. 2014;124:307-21.
- [CrossRef] [PubMed] [Google Scholar]
- Current status of probiotic and related health benefits. Appl Food Res. 2022;2:100185.
- [Google Scholar]
- Probiotic supplements: hope or hype? Front Microbiol. 2020;11:160.
- [CrossRef] [PubMed] [PubMed Central] [Google Scholar]
- A randomised, double-blind, placebo-controlled trial of a multi-strain probiotic in patients with asymptomatic ulcerative colitis and Crohn’s disease. Inflammopharmacology. 2019;27:465-73.
- [CrossRef] [PubMed] [PubMed Central] [Google Scholar]
- Effects of a probiotic product containing Bifidobacterium animalis subsp. Animalis IM386 and Lactobacillus plantarum MP2026 in lactose intolerant individuals: Randomized, placebo-controlled clinical trial. J Funct Foods. 2017;35:1-8.
- [Google Scholar]
- Amelioration of alcohol induced gastric ulcers through the administration of Lactobacillus plantarum APSulloc 331261 isolated from green tea. Front Microbiol. 2020;11:420.
- [CrossRef] [PubMed] [PubMed Central] [Google Scholar]
- Impact of probiotics and prebiotics targeting metabolic syndrome. J Funct Foods. 2020;64:64. 103666
- [Google Scholar]
- Chapter 27. Prospective role of prebiotics and probiotics in gut immunity. In: Bagchi D, Downs B, eds. Microbiome, immunity, digestive health and nutrition. Academic Press Elsevier; 2022b. p. :387-404.
- [Google Scholar]
- Role of probiotics in urogenital healthcare. J Mid Life Health. 2011;2:5-10.
- [CrossRef] [PubMed] [PubMed Central] [Google Scholar]
- Gut microbiota in human adults with type 2 diabetes differs from non-diabetic adults. Plos One. 2010;5:e9085.
- [CrossRef] [PubMed] [PubMed Central] [Google Scholar]